Understanding the Digital Signature Standard (DSS) A Deep Dive into X509 Certificate Requirements for Document Authentication in 2024
Understanding the Digital Signature Standard (DSS) A Deep Dive into X509 Certificate Requirements for Document Authentication in 2024 - X509 Certificate Structure and Key Components in DSS Authentication
Within the context of DSS authentication, the X509 certificate's structure is fundamental. It serves as a container for crucial data points that underpin secure digital interactions. Key elements within this structure include the certificate version, a unique serial number, details about the issuer and the subject (the entity being authenticated), the timeframe during which the certificate is valid, the public key used for verification, and the digital signature itself. This interconnected set of elements forms the basis for establishing trust and verifying the integrity of digital signatures, paving the way for reliable document authentication.
Crucially, the process relies on Certificate Authorities (CAs) as trusted third parties. CAs act as verifiers, confirming the identity of individuals or entities requesting certificates. Their involvement enhances the security of the entire system. Moreover, X509 certificates employ secure hashing algorithms, which play a vital role in ensuring the data's integrity and authenticity. This intricate web of components and processes not only facilitates secure document signing and verification but also reinforces cybersecurity across a range of digital applications, potentially beyond simply document authentication. While the concept is fundamental, questions about the longevity and evolution of the approach, in the face of emerging threats and technologies, remain open.
The X.509 standard, a cornerstone of public key infrastructure (PKI), has been around for quite some time, originally from 1988, and it's seen a series of updates. These revisions weren't just for the sake of change, they've been necessary to keep up with the increasing complexity and changing landscape of cryptography. It's interesting how this standard has managed to adapt and remains relevant.
Each X.509 certificate sports a unique serial number. Think of it like a social security number for certificates—ensuring that no two from the same Certificate Authority (CA) are the same. This feature is crucial for tracking and security purposes, although it's not bulletproof as we've seen.
X.509 certificates are central to PKI, a framework that relies on a hierarchical trust structure with multiple layers of CAs. This approach enables effective trust management within networks, but it also introduces complexity. Does this layered approach make things more secure, or are the multiple layers simply adding points of failure?
A key aspect of X.509 certificates is the digital signature produced by the CA using its private key. This signature provides a stamp of approval for the certificate, ensuring it's authentic and untampered. Furthermore, it acts as a defense mechanism against "man-in-the-middle" attacks. However, some researchers question whether the signatures are truly resilient.
Besides the public key, an X.509 certificate also stores a good amount of associated data like the owner's name, details about the CA that issued it, and the certificate's validity period. All of these pieces form the context and the lifespan of the certificate.
Certificates issued by a CA often have built-in expiration dates. This time-limited nature is designed to mitigate risks tied to compromised keys. But, it introduces the need for regular renewal, which can be a hassle. This ongoing need for renewal has also been a point of debate because it could become a burden or add complexity for certain use cases.
Revocation lists, such as the Certificate Revocation List (CRL) and Online Certificate Status Protocol (OCSP), are fundamental aspects of X.509. These are used to confirm if a certificate is still valid. If it's found to be revoked, it's treated as untrusted, thereby improving security. However, we still observe a significant number of issues with CRL and OCSP. Do we need a different kind of approach?
The Subject Alternative Name (SAN) field within X.509 certificates accommodates multiple domain names or IP addresses. This functionality allows a single certificate to secure multiple endpoints. It is becoming increasingly relevant in modern, complex environments with the rise of cloud computing and geographically dispersed infrastructure. It's noteworthy how the SAN field's versatility has evolved to address the modern infrastructure needs.
The X.509 standard utilizes ASN.1 (Abstract Syntax Notation One) to efficiently encode intricate data structures. ASN.1 enables easy communication between diverse systems and services, leading to improved interoperability across different networks. However, in light of new technologies and communication requirements, one could argue that the structure of ASN.1 could benefit from future revisions for broader applicability.
Recent innovations in X.509 have embraced modern cryptographic algorithms like Elliptic Curve Cryptography (ECC). ECC offers a great balance between security and efficiency, particularly in constrained environments. Using shorter keys to achieve the same level of security as traditional RSA algorithms leads to faster execution speeds. It's intriguing to examine how ECC has emerged as a viable option for enhanced performance.
Understanding the Digital Signature Standard (DSS) A Deep Dive into X509 Certificate Requirements for Document Authentication in 2024 - Updates to Digital Signature Standard FIPS 186-5 Requirements 2024
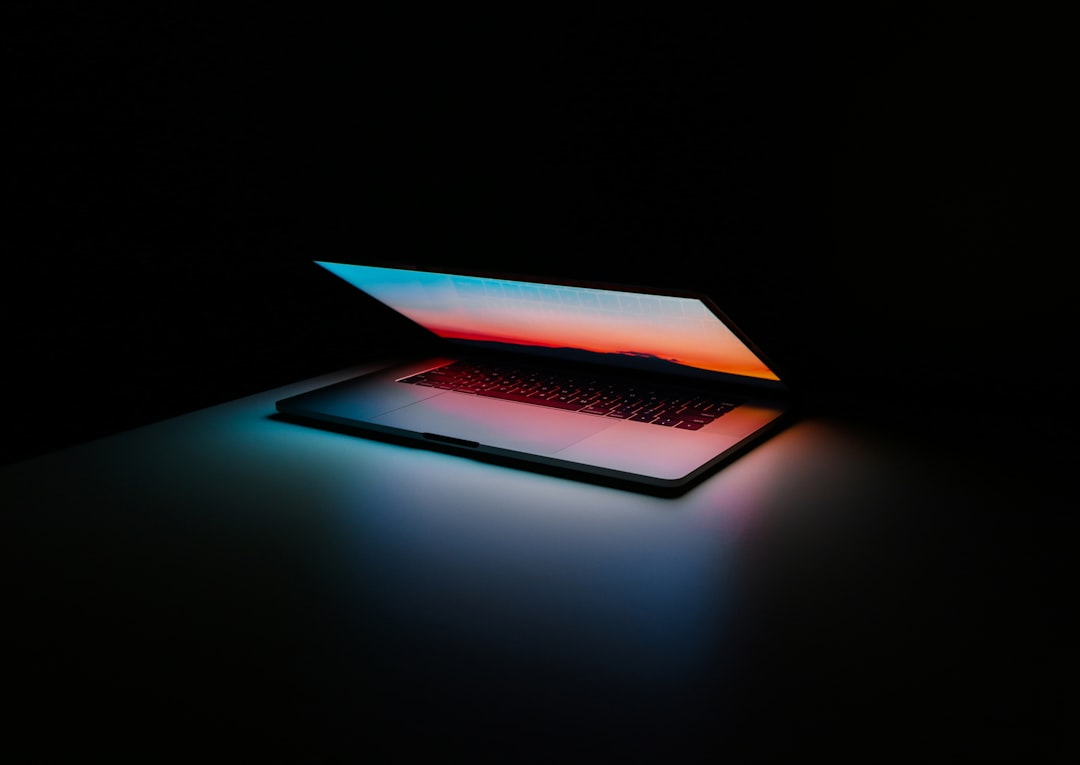
The Digital Signature Standard (DSS), specifically FIPS 186-5, has seen updates in 2024 aimed at strengthening the security of digital signatures. These updates are crucial as digital signatures are relied upon to verify the authenticity of signatories and prevent unauthorized data alterations. FIPS 186-5 provides a set of algorithms for creating these digital signatures, which are critical for secure digital interactions. Interestingly, it includes three different techniques for generating and verifying digital signatures, but the inclusion of a specific elliptic curve cryptography method, Schnorr 384, was deemed unnecessary due to limited demand.
While the current version of the DSS serves its purpose, NIST anticipates potential threats from quantum computing and is investigating and evaluating new public-key digital signature algorithms to improve security. The goal is to have a selection of algorithms ready for when quantum computers can crack current methods. In the future, there are plans for a new standard derived from FALCON, among other possibilities. The evolving nature of digital signatures in light of new computing paradigms necessitates continued refinement of standards like FIPS 186-5 to ensure the ongoing integrity and trustworthiness of digital transactions in a world increasingly reliant on electronic communications.
The latest version of the Digital Signature Standard, FIPS 186-5, released in 2024, introduces a set of updated requirements for digital signatures. These changes, aimed at improving cryptographic security, mean that organizations need to be aware of how these impact their current systems and security practices. One notable shift is the incorporation of new, more resilient algorithms designed to withstand the potential threat of quantum computing. This could require a fairly significant shift in how organizations manage cryptography, potentially necessitating the transition to entirely new frameworks – something that could add complexity to established systems relying on older methods.
The revised standard places great emphasis on robust key generation methods, a response to a growing number of key compromise incidents. This suggests that earlier approaches to key management may not have been as secure as previously thought. We're also seeing a push towards more advanced hash algorithms within the standard. This is likely due to rising concerns over potential hash collisions as computational power continues to increase, particularly with cloud computing. Organizations now need to carefully examine the entire lifecycle of their digital signature implementations, including the signing and verification processes. This will likely lead to a more stringent audit trail and possibly necessitate the adoption of new software tools to achieve compliance.
FIPS 186-5 also focuses on interoperability, aiming for greater compatibility between digital signature systems around the world. This makes sense, given the increasing globalization of digital interactions and commerce. The standard even incorporates guidance for mobile and IoT devices, recommending lightweight cryptography methods that prioritize security without sacrificing performance in environments with limited resources. This suggests an acknowledgement that digital signatures are becoming increasingly important in these types of devices.
The revision also addresses weaknesses in existing revocation practices by pushing for real-time revocation notification systems. This change moves away from the more traditional Certificate Revocation List (CRL) approach, which has been known to be vulnerable. It's a step towards enhancing security in real-time. Furthermore, the updated requirements mandate much more rigorous testing of digital signature implementations. This could mean that existing certifications might no longer guarantee sufficient protection against sophisticated attacks. It certainly raises questions about past practices and certifications.
Finally, and importantly, FIPS 186-5 acknowledges the crucial role of user education and awareness. This shows a more holistic approach to digital signature security that recognizes the importance of human factors alongside technological advancements. This signals a broader understanding that security relies on both technical implementation and end-user awareness and preparedness. Overall, the updated standards present a mix of anticipated changes and surprising adjustments in how we handle digital signatures, pointing toward the continuous evolution of digital security in the face of emerging threats.
Understanding the Digital Signature Standard (DSS) A Deep Dive into X509 Certificate Requirements for Document Authentication in 2024 - Certificate Authority Role and Trust Chain Validation Methods
Certificate Authorities (CAs) act as trusted intermediaries, issuing digital certificates that verify the identities of websites, organizations, or individuals involved in online interactions. They essentially serve as the guarantors of trust within digital communications, ensuring that parties interacting online are who they claim to be. The process relies on a chain of certificates, where each certificate builds on the trust established by the preceding one, starting from a root Certificate Authority down to the entity being authenticated (the "end entity"). This structured trust model, however, introduces complexity, especially when considering the potential weaknesses at each link in the chain. The validity and reliability of the entire system depend on each CA diligently fulfilling its role and maintaining the security of its operations.
But the ongoing landscape of digital security is far from static. The threat environment continuously evolves, with new attack vectors and vulnerabilities emerging. These changes raise important questions about how effective traditional trust chain validation methods remain in light of modern threats and whether updates are necessary to guarantee continued security in an increasingly complex digital landscape. The reliance on a hierarchy of trust raises concerns regarding potential single points of failure or instances where the trust model might be circumvented. As reliance on digital certificates and digital signature methods continues to increase, a robust, flexible, and constantly updated approach to validation is critical to maintain confidence and safety in the online world.
Certificate Authorities (CAs) play a crucial role in establishing trust online by issuing digital certificates. These certificates, essentially digital IDs, vouch for the identity of websites, organizations, or servers. However, the reliance on CAs brings about challenges. For example, imagine if a core CA, the foundation of this trust system, was compromised—it could cascade into a massive security breach across the entire network of certificates it's responsible for. This highlights a potential vulnerability in the very structure that underpins trust.
The X.509 standard allows for flexibility through what's known as delegated path validation. This means that trust can be passed down the line, between intermediate CAs. While this approach is useful in complicated environments, it can introduce new avenues for attacks if any intermediary CA within the chain isn't trustworthy. It's a bit like a game of telephone; each time the trust message passes through a hand, there's a chance for errors or deliberate alterations.
Interestingly, there's a push towards shorter-lived certificates, often lasting only 90 days. While this limits the impact of compromised keys, it adds operational burden—the need for constant certificate renewal, potentially leading to complications and possible downtimes if not handled precisely. Maintaining this system can be quite demanding.
The traditional methods for revoking certificates—things like the Certificate Revocation List (CRL) and Online Certificate Status Protocol (OCSP)—are still struggling with issues like latency and accessibility. This means systems could unknowingly accept a certificate that has actually been revoked, making these security features less effective than intended.
Recently, there's been interest in blending X.509 certificates with blockchain technologies. This potentially could introduce a new layer of transparency and immutability, making it harder to manipulate certificate data. But, like any new approach, it's important to ensure the introduction of blockchain doesn't inadvertently bring its own vulnerabilities.
The looming threat of quantum computing is also casting a shadow over current cryptographic practices. We're on the cusp of a shift towards post-quantum cryptographic algorithms. This will reshape future certificates and introduce a whole new level of complexity into how we think about cryptography.
Unfortunately, a significant number of security incidents have been linked to basic user mistakes in configuring certificate settings. This highlights the continued need for better tools and education to prevent human error.
The field of cryptography is continuously evolving. As old algorithms are phased out in favor of newer, more robust alternatives, this can lead to sudden shifts in how organizations need to handle cryptography. Adaptability and staying current with standards are crucial in this field to avoid potential security gaps.
The certificate chain validation process itself is surprisingly intricate. There are many things involved in validating a certificate chain, like checking cryptographic signatures, verifying names, and understanding usage policies. This complexity can easily lead to errors, particularly in environments with varied policies and practices. It highlights the need for comprehensive and consistent approaches to ensuring a strong foundation for trust.
It's evident that while X.509 certificates form the backbone of online trust, the inherent complexity and vulnerabilities necessitate a constant vigilance in adapting to new technologies and threats. We need to find a balance between innovation and security, ensuring that the mechanisms that undergird trust in our digital interactions remain robust and reliable.
Understanding the Digital Signature Standard (DSS) A Deep Dive into X509 Certificate Requirements for Document Authentication in 2024 - RSA vs ECDSA Digital Signature Algorithms Performance Analysis
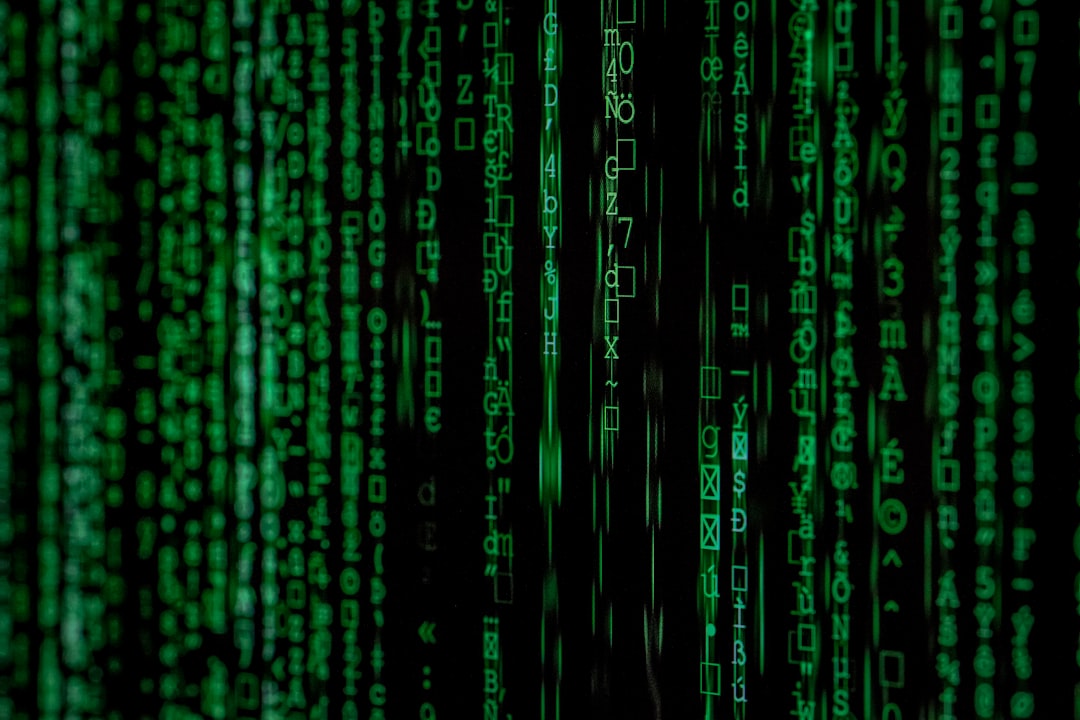
When comparing RSA and ECDSA in terms of performance, we find some key distinctions. ECDSA, with its reliance on elliptic curve cryptography, can achieve similar levels of security with much smaller key sizes compared to RSA. This translates to faster key generation, signature creation, and verification, particularly beneficial for devices with limited computing power like smartphones or embedded systems. In contrast, RSA, while still widely used, requires larger keys for equivalent security, potentially leading to slower operations. This performance difference becomes increasingly relevant as digital signatures become more commonplace in document authentication and other areas. The ongoing trend toward adopting smaller, more efficient algorithms like ECDSA hints at a shift in preferred practices within the field, driven by the need for optimized security and performance in a fast-paced digital environment. Staying ahead of both technological advancements and emerging security threats demands a continuous reevaluation of the cryptographic methods we use to ensure secure digital interactions.
RSA and ECDSA are two prominent digital signature algorithms within the Digital Signature Standard (DSS). While both serve the same core purpose of verifying data integrity and authenticity, they differ significantly in their performance characteristics. ECDSA, which relies on elliptic curve cryptography, generally boasts faster key generation, signature creation, and verification speeds compared to RSA. This stems from its use of smaller key sizes to achieve similar security levels. For instance, a 224-bit ECDSA key offers comparable security to a 2048-bit RSA key, leading to reduced storage and bandwidth requirements, and making ECDSA particularly suitable for resource-constrained environments like mobile devices or IoT applications.
However, this advantage comes at a cost. The intricate mathematics behind ECDSA can present challenges in implementation and debugging, requiring specialist knowledge to ensure security. Moreover, while ECDSA has several performance benefits, the transition from RSA has been gradual, with many legacy systems still favoring RSA. This creates a blended environment where compatibility issues can arise. Furthermore, both algorithms are facing scrutiny in the context of quantum computing, which could potentially crack the underlying cryptographic schemes. This creates uncertainty regarding the long-term viability of both.
One interesting aspect is that for small data payloads, RSA signatures with message recovery can be more compact than ECDSA signatures. However, as the size of the data increases, this advantage lessens. It’s also worth noting that RSA, having been around longer, has wider adoption and established implementations. It’s interesting to see how the push for more resilient algorithms within updated standards like FIPS 186-5 suggests a movement away from RSA towards ECDSA. This shift potentially leads to a more diverse and adaptable security landscape, but it also requires organizations to carefully consider compatibility, implementation complexities, and the future of cryptography in light of looming quantum computing threats.
It’s clear that the choice between RSA and ECDSA hinges on specific circumstances and trade-offs between performance, security, and implementation complexities. As quantum-resistant algorithms gain prominence, we'll likely see a more nuanced shift in the utilization of RSA and ECDSA. The future will likely involve further refinement and potentially a combination of techniques that leverage the strengths of both algorithm types to meet the increasingly demanding needs of secure digital communication and data exchange.
Understanding the Digital Signature Standard (DSS) A Deep Dive into X509 Certificate Requirements for Document Authentication in 2024 - Technical Implementation Steps for Document Signing with X509
Implementing document signing with X.509 certificates involves a series of steps to ensure proper authentication. First, you need to create a key pair, a public and private key, which is the foundation for creating a digital signature. The digital signature itself is created by taking the document, hashing it, and then encrypting the resulting hash with the signer's private key. This process ensures both data integrity and that the signer can't later deny signing. Then, the signed document is sent to the recipient along with the X.509 certificate. This certificate contains the signer's public key and identity information. When the recipient receives the document, they can verify the signature by decrypting it with the signer's public key. This confirms the authenticity of the signer and the integrity of the document. Each stage of this process underscores the need to maintain robust cryptography, particularly as organizations increasingly adopt X.509 for document-related security, especially given the changing nature of cyber threats.
X.509 certificates offer a granular approach to security by allowing for fine-tuning of permissions on a per-certificate basis. For example, you can control which encryption methods a certificate can use or specify which applications should trust it. This level of control can greatly improve security, but it also leads to a more complex certificate management landscape.
The effectiveness of digital signatures is directly related to the length of the public key employed. While longer keys usually mean better security, the size of these keys needs careful management. We see ECDSA offering comparable security with much smaller key sizes than RSA. However, this performance optimization needs to be weighed carefully, especially in environments where computational resources are scarce, such as mobile devices.
Revocation mechanisms like CRLs and OCSP are designed to improve security by identifying and invalidating compromised certificates. But these mechanisms come with their own set of complexities and vulnerabilities. In practice, they can introduce latency and permission conflicts, especially when there's network instability that hinders rapid verification of the revocation status.
We're seeing a growing interest in cryptographic hybrids that combine established techniques like RSA with newer, elliptic curve methods. These hybrids offer a more resilient approach to security against emerging threats, while also enabling organizations to more gradually transition away from legacy systems.
While X.509 and its associated technologies are highly sophisticated, a significant number of security breaches occur due to simple user errors. This highlights the critical need for strong user education and training programs as a fundamental part of any digital signature strategy.
The latest standards are pushing for real-time certificate revocation notifications to replace the more traditional CRL approach. However, these new systems demand major infrastructure changes that can be quite challenging to implement.
Modern X.509 implementations strongly emphasize interoperability across diverse systems. This increased focus reflects the need for consistent and smooth communication between various digital signature frameworks, particularly as more businesses become globalized.
ASN.1, the standard used to encode data in X.509 certificates, is a very powerful tool for handling complex data structures. However, it's also rather complex and can be prone to misinterpretations. This makes it important to carefully validate any implementations that rely on this standard.
The ongoing development of post-quantum cryptographic algorithms has become a focal point as the possibility of quantum computing becomes closer. Researchers are actively exploring alternatives to currently used methods, like RSA and ECDSA, to safeguard against potential future attacks. This work is vital to maintaining the long-term effectiveness of secure digital communication.
There's a clear trend in the evolution of digital signature methods, moving from widely-used traditional methods such as RSA toward more modern algorithms like ECDSA, driven by factors like performance improvements and efficiency. This movement reveals a desire to optimize security practices to match the continuously evolving capabilities of computation.
Understanding the Digital Signature Standard (DSS) A Deep Dive into X509 Certificate Requirements for Document Authentication in 2024 - Security Vulnerabilities and Mitigation Strategies in DSS
The Digital Signature Standard (DSS), while designed to enhance document security, isn't immune to vulnerabilities. Weaknesses in traditional methods, like key management practices, are becoming increasingly apparent as cyber threats become more sophisticated. The need for more rigorous key generation and verification techniques is more pressing than ever. Furthermore, the potential impact of quantum computing on current cryptographic methods is a growing concern, demanding exploration of new, post-quantum signature algorithms to secure digital signatures in the long run. In response, updates to the standard, specifically FIPS 186-5, are pushing for real-time certificate revocation processes and improved interoperability between various systems. This reflects a broader shift towards a more holistic approach to digital signature security. Beyond the technical aspects, user education and training are essential to mitigating vulnerabilities caused by simple user errors. In essence, building secure systems necessitates both robust technological foundations and a culture of security awareness among users.
The Digital Signature Standard (DSS), defined in FIPS 186-5, is a US government standard that establishes a set of algorithms for creating digital signatures. Designed by the NSA, DSS aims to authenticate electronic documents in a manner akin to traditional handwritten signatures, ensuring both the identity of the signer and the data's integrity. DSS leverages Secure Hash Algorithms (SHA) as part of the signature creation process, generating a unique bitstring that represents the signature itself. The receiver of the signed data can use this bitstring, along with associated certificates, to demonstrate authenticity and integrity to any third party, thus upholding the trust in the signed document.
It's crucial to note that DSS primarily focuses on the conceptual aspects and the broader technological considerations of digital signatures, rather than diving deep into the specific mathematical nuances of the cryptographic techniques. DSS sets a minimum standard for the creation and verification of digital signatures to ensure consistency across applications and systems. While DSS and its associated methods (like DSA) are mandated for certain government and commercial uses, adherence to the standard itself creates a complex landscape that has potential vulnerabilities. For instance, many implementations rely on traditional cryptographic methods that are being increasingly scrutinized as computing power grows and new attack vectors emerge.
Ongoing concerns around certificate lifespans, especially given the frequent push for shorter validity periods like 90 days, present a challenge. Certificate management in general can become quite complex, adding overhead for organizations. Moreover, certificate revocation systems have some well-documented weaknesses. Traditional CRLs, for example, often suffer from delays and occasional unreliability. The inherent complexity of the X.509 standard itself can lead to incompatibility problems when updates or transitions in cryptography occur, posing risks if proper interoperability isn't a primary design goal. Human error in configurations remains a consistent issue, making education and user awareness critically important aspects of a robust security posture.
We're in a period where the impact of future quantum computing capabilities is looming large. Existing algorithms that are currently considered secure might not withstand attacks by quantum computers in the future. This reality puts the necessity of studying and implementing quantum-resistant cryptographic methods into sharper focus. Further, the concept of a 'delegated path' in the X.509 trust model, though flexible, can be a source of vulnerability if proper validation practices aren't consistently maintained across the intermediate certificate authorities. While DSS has been designed to be adaptable, organizations often need to balance this adaptability with compliance and the associated costs of adopting updated standards like FIPS 186-5. This includes accounting for new training procedures, software upgrades, and the need for periodic audits. In essence, cryptography is in a constant state of evolution, and the threat landscape is becoming more complex, requiring us to adopt not just technological updates, but also an adaptable mindset.
ASN.1, a core component of X.509, provides a highly versatile means for encoding certificate information, but this versatility can introduce complexity that leads to errors if implementations aren't rigorously validated. In short, the DSS serves a fundamental need in the digital world, but there are areas that require both technical and procedural vigilance. This includes an ongoing reevaluation of the methods used, an adaptability to both standards and attacks, and an emphasis on training in a field where security is a continuous pursuit, not a one-time fix.
More Posts from legalpdf.io: